Definition of Resistance Temperature Detector (RTD)
RTD is an abbreviation for Resistance Temperature Detector. In the following, we will introduce RTD from five aspects.
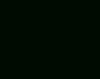
Measurement Principle
RTD measures temperature by utilizing the property of metal resistance increasing with temperature. Why do metals have such a characteristic? It is because metals have a small number of outer electrons and are prone to lose electrons.
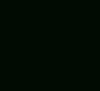
When a large number of metal atoms gather, most of them lose their valence electrons (outer electrons).
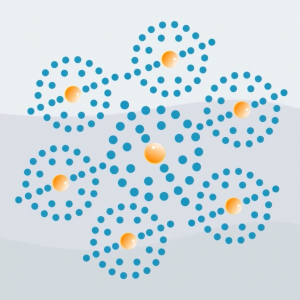
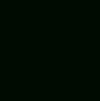
At this point, positive ions will arrange themselves in a certain geometric form and perform high-frequency thermal vibration at fixed positions, while the lost electrons become free electrons and move among the positive ions, resulting in good electrical conductivity of the metal.
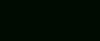
However, when the temperature of the metal rises, the thermal vibration amplitude between positive ions increases, hindering the movement of free electrons, leading to an increase in the metal’s resistance.
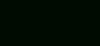
Common Types
Not all metals are suitable for use as measuring resistance. After screening, platinum, copper, and nickel are chosen as the materials for RTD.
Platinum has stable and corrosion-resistant characteristics, and it does not undergo physical or chemical changes due to high or low temperatures. Therefore, platinum RTD is the most accurate and stable method for measuring temperature and is widely used in industrial production. Common models include PT100, PT500, PT1000, etc.
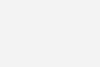
Nickel is a hard and ductile metal that is relatively corrosion-resistant. However, prolonged use of nickel metal accelerates aging and affects measurement accuracy, limiting the application range of nickel RTD.
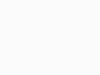
Copper is a relatively soft metal with good ductility and conductivity. At a certain temperature, the linearity of copper’s resistance-temperature relationship is good. However, copper undergoes oxidation at high temperatures, affecting accuracy, so copper RTD is more commonly used in low-temperature scenarios. Common models include Cu50, Cu100.

What do these RTD model numbers mean? Taking PT100 as an example, PT indicates that it is a platinum resistance, and 100 indicates that its resistance value at 0℃ is 100Ω. Other model numbers follow a similar naming convention.
Advantages of RTD
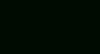
From the chart, it can be observed that RTD has the best resistance-temperature linearity.
The table compares three types of sensors: resistance temperature detectors (RTD), thermistors, and thermocouples. It can be seen that RTD has a significant advantage in measuring temperatures at medium and low levels. For high-temperature measurements, thermocouples perform better. At certain temperatures, high-precision thermistors are more suitable. Although RTD has good linearity, to obtain relatively accurate temperature values, we typically refer to calibration tables. The following chart shows the PT100 RTD calibration table.
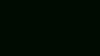
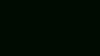
Measurement Method
Now that we understand the measurement principle of RTD, let’s discuss how RTD is actually measured.
When measuring resistance, we first think of using Ohm’s Law: R = U/I.
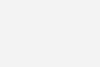
When measuring resistances with relatively low values, a constant current source is commonly used as the excitation, and then the voltage across the resistance is measured to calculate its resistance value. The maximum excitation current used for RTD is 5mA, so typically, 1mA or even smaller currents are used as the excitation. The specific measurement methods can be categorized into three forms: two-wire, three-wire, and four-wire configurations.
The circuit diagram for two-wire measurement is similar to the one above, but there is an impact from lead resistance in practical measurements, as shown below:
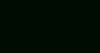

Where ρ is the resistivity of the conductor, l is the length of the conductor, and s is the cross-sectional area of the conductor.
Now, let’s try to calculate the resistance of the lead wire. Assuming a PT100 with a wire length of 1 meter is used for temperature measurement, and the wire has a radius of 0.2 mm. The resistivity of the wire is known to be 1.74×10-8.
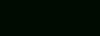
The resistance of a single wire can be calculated as 0.14Ω. For two-wire measurement, two wires are used, resulting in a total resistance error of 0.28Ω. When the measured resistance is 111.29Ω, referring to the PT100 calibration table, we can deduce that the temperature is 29℃. However, due to the resistance error caused by the lead wire, the actual resistance value is 111.01Ω, and the actual temperature is calculated to be 28℃.
Therefore, the lead wire introduces an error of 1℃, and this error will increase with longer lead wires. Now, let’s consider four-wire measurement:
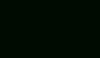
The four-wire method directly measures the voltage across the resistance by introducing two wires, thereby eliminating the effect of lead resistance and providing the most accurate temperature measurement. However, since it adds two additional wires, it increases the implementation difficulty and cost, so the four-wire configuration is mainly used in high-precision laboratory experiments. The three-wire configuration strikes a balance between cost and measurement accuracy, making it a popular choice among users.
In the three-wire method, an additional current source is added to compensate for the voltage produced by lead resistance, thus controlling the error within a reasonable range. Here’s a simple explanation: The voltage value V is obtained by subtracting V1 from V2. Let’s analyze the direction of current I1. I1 flows out of current source 1, passes through lead resistance 1, goes through the RTD resistance, and finally flows through lead resistance 3.
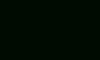
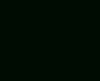
This method ensures that the measured voltage value is the voltage across the RTD, effectively eliminating the impact of lead resistance.
Temperature Acquisition
Next, we will use a PT100 sensor to monitor the temperature variations during the use of a mobile phone. We will employ a three-wire PT100 thin-film resistance temperature detector (RTD) to measure the temperature of the mobile phone. This type of RTD is a new generation temperature measurement and regulation sensor known for its fast response time, high accuracy, compact size, and suitability for measuring temperatures of liquids, gases, and solid surfaces.
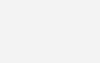
If using a stainless steel sheathed RTD, the resistance body and leads are inserted into a stainless steel sheath, which serves as protection and is the most common packaging method (as shown in the figure below).
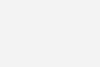
During temperature measurement, the sensing end of the RTD is placed in contact with the object being measured. We connect the RTD to the M2111 RTD temperature acquisition module and establish a connection to a computer through a 485 serial port converter. This allows us to display real-time temperature data on the computer.
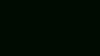
The figure above shows the connected setup. We then place the resistance temperature sensor near the mobile phone’s camera. After configuring the relevant parameters in the acquisition software, we begin the test while playing a game to observe how much the phone’s temperature increases during a game session.
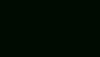
After completing the test, analyzing the waveform reveals that several decreasing intervals occurred due to placing the phone on a relatively cool surface, while the overall trend shows an upward temperature increase.
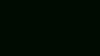
By inspecting the stored CSV file, we can view the temperature data collected by the sensor at each moment.
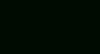
Returning to the waveform graph and zooming in on the y-axis, we can observe that the current phone temperature is 37.7°C, and its initial temperature was 32.4°C before starting the game. The game session lasted approximately 15 minutes. Based on this data, we can estimate that the phone’s temperature increased by approximately 0.35°C per minute during gameplay. However, please note that this is just an estimation, and various factors might not have been accounted for.
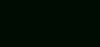
This concludes the entire process of using the RTD sensor for temperature measurement. Further information about software usage and module details can be found on our website’s relevant pages.